Results from Fermilab’s Muon g-2 experiment strengthen evidence of new physics
- Posted By
10Pointer
- Categories
Science & Technology
- Published
13th Apr, 2021
-
-
Context
In the latest development, scientists in the field of particle physics at “Fermilab” in Batavia, Illinois announced that they have found evidence that a tiny subatomic particle is disobeying the known laws of physics.
-
Background
- Fermilab is one of the world’s finest laboratories dedicated to studying fundamental questions.
- It started in 2001, at Brookhaven National Lab, where the physicist found that fundamental particles called muons weren’t behaving the way they were supposed to in the presence of an external magnetic field.
- It was called the g-2 anomaly (after a number called the gyromagnetic factor).
- Twenty years after an apparent anomaly in the behavior of elementary particles the Physicists at Fermi National Accelerator Laboratory found that muons (elementary particles similar to electrons) wobbled more than expected while whipping around a magnetized ring.
- The experiment was called Muon g–2(g minus two)was performed at the
-
Analysis
What is the Standard Model?
The biggest and the most succinct summarizing question is ‘Why is there something, rather than nothing? We don’t have appropriate explanations for any of those questions. But because of centuries of scientific effort, we have made a lot of progress, and is known to us that the universe began in an enormous explosion, evidently called the “Big Bang”. We have also devised a detailed if incomplete, understanding of the rules that govern subatomic matter. That theory is called the “Standard Model”.
- The Standard Model is a well-established and universally accepted theory that predicts the behavior of the building blocks of the universe.
- The standard model of particle physics explains the subatomic world of molecules and atoms as being built of twelve even smaller particles called quarks and “Leptons”.
- Those particles are the smallest known building blocks of the cosmos and they are governed by four forces, called strong and weak nuclear forces, electromagnetism, and gravity.
- Finally, a ghostly field called the Higgs field gives mass to some of those particles and completes the theory.
- It lays out the rules for six types of quarks, “six leptons”(muon is one among them), the Higgs boson, three fundamental forces (gravitational force is not considered as the fundamental force), and how the subatomic particles behave in the vicinity of external electromagnetic forces.
-
What is muon?
- The muon was discovered as a constituent of “cosmic-ray particle showers” in 1936.
- The muon is one of the It is similar to the electron, but 207 times heavier, and much more unstable, surviving for a fraction of a second.
- The muon is an elementary subatomic particle. It is a negatively charged particle and with half spin. Its symbol is "μ-".
- It has two forms; one is called the negatively charged muon and the other is its positively charged antiparticle.
- Considering it to be 207 times heavier than the electron, it is also known as the ‘fat electron’. It is produced in significant numbers at the Fermilab experiments and occurs naturally in cosmic ray showers.
- A muon is relatively unstable as compared to the electron, with a lifetime of only 2.2 microseconds before it decays by the weak force into an electron and two kinds of neutrinos.
- Muons are extremely penetrating and can travel thousands of meters below the Earth’s surface.
|
-
What is this experiment trying to find out?
- It measured a quantity relating to the muon(g-factor), which was a follow-up of a previous experiment at Brookhaven National Laboratory, under the US Department of Energy.
- In 2001, the Brookhaven experiment came up with results that did not identically match predictions by the Standard Model.
- The Muon g–2 experiment measured this quantity with greater accuracy as the massive improvements were made in the detector this time.
- The whole experiment revolved around finding out whether the discrepancy would persist, or whether the new results would be closer to predictions (theoretical). And it turns out to be, a discrepancy once again, although smaller.
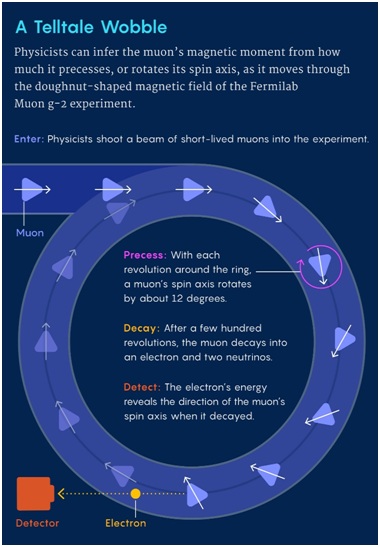
-
What quantity was measured in the experiment?
- Similar to the electrons, muons too exhibit properties like that of a tiny internal magnet.
- In a strong magnetic field, the direction of the muon’s magnet precession, or wobbles, just like the axis of a spinning top. The strength of the internal magnet determines the rate that the muon’s precession in an external magnetic field and is described by a number that physicists call the g-factor.
- This value of the g-factor is known to be close to 2, so scientists are measuring the deviation from 2. Hence the name of the experiment is g–2.
- Using the Standard Model, the precise value of the “g-factor” can be determined. In the g–2 experiment, scientists used high-precision instruments to measure the g-factor. They generated muons and then circulated them using a large magnet. The muons also interacted with another subatomic particle “popping in and out of existence”. These interactions have a definite effect on the value of the g-factor, resulting in muons wobbling slightly faster or slightly slower.
- The standard model is also able to calculate this deviation (this is called anomalous magnetic moment), too, with great precision. But if the interacting particles contain additional forces or particles that are not accounted for by the Standard Model, g-factor further reading will diverge.
-
What were the outcomes of the experiment?
- The results, while diverging from the Standard Model prediction, strongly in agreement with the Brookhaven results.
- The theoretical and experimental values for the muon are:
g-factor: 2.00233183620
anomalous magnetic moment: 0.00116591810
|
- The combined results from the (Brookhaven and Fermilab) results are:
g-factor: 2.00233184122
anomalous magnetic moment: 0.00116592061.
|
“This is probably suggesting that there are forms of matter and energy vital to the nature and evolution of the universe that are not yet known to science.”
-
What does this mean?
- The results hint at the existence of unknown interactions between the muon and the magnetic field that could possibly involve new particles or forces.
- The experimental data of Fermilab researchers suggests that the difference has grown to a level quantified as “4.2 sigmas,” well on the way to the benchmark of five-sigma level which is required to claim a discovery. While it may not be enough to claim a discovery but it is less likely to be a fluke.
A five-sigma result is considered the gold standard for significance, which means that the results are having about a one-in-a-million chance that the findings are just a result of random variations; whereas a six-sigma translates to one chance in a half-billion that the result is a random fluke. |
- The discrepancy strongly suggests that unknown particles (arising due to the uncertainty principle) are giving muons an extra push resulting in a result that diverges from the theoretical results.
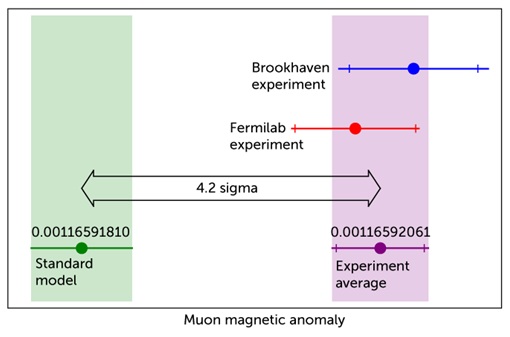
-
Conclusion
This is strong evidence that the muon is sensitive to something that is not a part of the standard model of particle physics. It is, however, not the last word in opening up the road to new physics. Although these first results are telling us that there is an intriguing difference with the Standard Model, we will learn much more in the next couple of years.